Assessment of biochar quality and agronomic efficiency produced from rice-husk and saw-dust at different temperature regimes
Abstract
Declining soil fertility and the limited use of sustainable soil organic amendments has resulted in reduced crop productivity in Nepal. This study assessed biochar produced from rice husk and sawdust at three different pyrolysis temperatures (200°C, 400°C, and 600°C), characterized their properties and applied them as soil amendments to test their agronomic effect on kidney bean production. The highest biochar yields were achieved at lower pyrolysis temperatures (200°C) for both rice husk (40%) and sawdust (38.4%). Ash content was significantly higher in rice husk (33.6%) compared to sawdust biochar (5.8%) across all temperatures. Sawdust biochar had higher volatile matter (91%) than in rice husk biochar (61.5%). The fixed carbon content was greater at 200°C and 400°C for both rice husk and sawdust biochar. FT-IR result showed significant loss of aromatic groups with increasing temperature. Biochar from all three temperatures was then used in a pot experiment to grow kidney beans and assess their agronomic effects. Seven treatments were used: control (CK), rice husk biochar at 200°C (RH200), 400°C (RH400), and 600°C (RH600), sawdust at 200°C (SD200), 400°C (SD400), and 600°C (SD600) following a completely randomized design with 3 replications per treatment. Cattle manure was applied uniformly (25 t ha-1) across all treatments, including the control. Over 50 days, SD400 resulted in the tallest plants, SD600 produced the thickest stem and RH600 had the highest number of leaves. Biochar applications showed significantly higher fruit weight and counts, which was on average 24 % higher than the control, with no significant differences between rice husk and saw dust biochar at three different temperatures. The study suggests that high quality biochar can be produced from both rice husk and saw dust and its application boost legume yields, which is crucial for enhancing country’s nutritional and food security.
Keywords:
Biochar, Pyrolysis temperature, FTIR, ProductivityDownloads
References
Acharya, N., Vista, S. P., Shrestha, S., Neupane, N., & Pandit, N. R. (2023). Potential of Biochar-Based Organic Fertilizers on Increasing Soil Fertility, Available Nutrients, and Okra Productivity in Slightly Acidic Sandy Loam Soil. Nitrogen, 4(1), Article 1. https://doi.org/10.3390/nitrogen4010001
Adekanye, T., Dada, O., & Kolapo, J. (2022). Pyrolysis of maize cob at different temperatures for biochar production: Proximate, ultimate and spectroscopic characterisation. Research in Agricultural Engineering, 68(1), 27–34. https://doi.org/10.17221/106/2020-RAE
Adeniyi, A. G., Adeyanju, C. A., Emenike, E. C., Otoikhian, S. K., Ogunniyi, S., Iwuozor, K. O., & Raji, A. A. (2022). Thermal energy recovery and valorisation of Delonix regia stem for biochar production. Environmental Challenges, 9, 100630. https://doi.org/10.1016/j.envc.2022.100630
Adhikari, S., Timms, W., & Mahmud, M. A. P. (2022). Optimising water holding capacity and hydrophobicity of biochar for soil amendment – A review. Science of The Total Environment, 851, 158043. https://doi.org/10.1016/j.scitotenv.2022.158043
Ahmee, K., & Yakob, A. (2021). Role of Bio Char on the Amelioration of Soil Acidity. Agrotechnology, Vol.10, No: 212.
Akhtar, S. S., Li, G., Andersen, M. N., & Liu, F. (2014). Biochar enhances yield and quality of tomato under reduced irrigation. Agricultural Water Management, 138, 37–44. https://doi.org/10.1016/j.agwat.2014.02.016
Ali, I., Ullah, S., He, L., Zhao, Q., Iqbal, A., Wei, S., Shah, T., Ali, N., Bo, Y., Adnan, M., Amanullah, & Jiang, L. (2020). Combined application of biochar and nitrogen fertilizer improves rice yield, microbial activity and N-metabolism in a pot experiment. PeerJ, 8, e10311. https://doi.org/10.7717/peerj.10311
Armynah, B., Atika, Djafar, Z., Piarah, W. H., & Tahir, D. (2018). Analysis of Chemical and Physical Properties of Biochar from Rice Husk Biomass. Journal of Physics: Conference Series, 979, 012038. https://doi.org/10.1088/1742-6596/979/1/012038
Azeem, M., Hayat, R., Hussain, Q., Ahmed, M., Pan, G., Ibrahim Tahir, M., Imran, M., Irfan, M., & Mehmood-ul-Hassan. (2019). Biochar improves soil quality and N2-fixation and reduces net ecosystem CO2 exchange in a dryland legume-cereal cropping system. Soil and Tillage Research, 186, 172–182. https://doi.org/10.1016/j.still.2018.10.007
Bruun, E. W., Ambus, P., Egsgaard, H., & Hauggaard-Nielsen, H. (2012). Effects of slow and fast pyrolysis biochar on soil C and N turnover dynamics. Soil Biology and Biochemistry, 46, 73–79. https://doi.org/10.1016/j.soilbio.2011.11.019
Bruun, S., Jensen, E. S., & Jensen, L. S. (2008). Microbial mineralization and assimilation of black carbon: Dependency on degree of thermal alteration. Organic Geochemistry, 39(7), 839–845. https://doi.org/10.1016/j.orggeochem.2008.04.020
Chen, D., Liu, D., Zhang, H., Chen, Y., & Li, Q. (2015). Bamboo pyrolysis using TG–FTIR and a lab-scale reactor: Analysis of pyrolysis behavior, product properties, and carbon and energy yields. Fuel, 148, 79–86. https://doi.org/10.1016/j.fuel.2015.01.092
Chi, N. T. L., Anto, S., Ahamed, T. S., Kumar, S. S., Shanmugam, S., Samuel, M. S., Mathimani, T., Brindhadevi, K., & Pugazhendhi, A. (2021). A review on biochar production techniques and biochar based catalyst for biofuel production from algae. Fuel, 287, 119411. https://doi.org/10.1016/j.fuel.2020.119411
Cornelissen, G., Jubaedah, Nurida, N. L., Hale, S. E., Martinsen, V., Silvani, L., & Mulder, J. (2018). Fading positive effect of biochar on crop yield and soil acidity during five growth seasons in an Indonesian Ultisol. Science of The Total Environment, 634, 561–568. https://doi.org/10.1016/j.scitotenv.2018.03.380
Cornelissen, G., Martinsen, V., Shitumbanuma, V., Alling, V., Breedveld, G., Rutherford, D., Sparrevik, M., Hale, S., Obia, A., & Mulder, J. (2013). Biochar Effect on Maize Yield and Soil Characteristics in Five Conservation Farming Sites in Zambia. Agronomy, 3(2), 256–274. https://doi.org/10.3390/agronomy3020256
Cornelissen, G., Pandit, N. R., Taylor, P., Pandit, B. H., Sparrevik, M., & Schmidt, H. P. (2016). Emissions and Char Quality of Flame-Curtain “Kon Tiki” Kilns for Farmer-Scale Charcoal/Biochar Production. PLOS ONE, 11(5), e0154617. https://doi.org/10.1371/journal.pone.0154617
Crombie, K., Mašek, O., Sohi, S. P., Brownsort, P., & Cross, A. (2013). The effect of pyrolysis conditions on biochar stability as determined by three methods. GCB Bioenergy, 5(2), 122–131. https://doi.org/10.1111/gcbb.12030
Dahal, S., Vista, S. P., Khatri, M., & Pandit, N. R. (2021). Effect of biochar blended organic fertilizers on soil fertility, radish productivity and farm income in Nepal. Archives of Agriculture and Environmental Science, 6(4), 416–425. https://doi.org/10.26832/24566632.2021.060402
Dume, B., Berecha, G., & Tulu, S. (2015). Characterization of Biochar Produced at Different Temperatures and its Effect on Acidic Nitosol of Jimma, Southwest Ethiopia. International Journal of Soil Science, 10(2), 63–73. https://doi.org/10.3923/ijss.2015.63.73
Elkhlifi, Z., Iftikhar, J., Sarraf, M., Ali, B., Saleem, M. H., Ibranshahib, I., Bispo, M. D., Meili, L., Ercisli, S., Torun Kayabasi, E., Alemzadeh Ansari, N., Hegedűsová, A., & Chen, Z. (2023). Potential Role of Biochar on Capturing Soil Nutrients, Carbon Sequestration and Managing Environmental Challenges: A Review. Sustainability, 15(3), 2527. https://doi.org/10.3390/su15032527
Etminani, A., Mohammadi, K., & Saberali, S. F. (2021). Effect of organic and inorganic amendments on growth indices and seed yield of red kidney bean (Phaseolus vulgaris) in competition with Amaranthus retroflexus. Journal of Plant Nutrition, 44(3), 421–437. https://doi.org/10.1080/01904167.2020.1822398
Farhangi-Abriz, S., Torabian, S., Qin, R., Noulas, C., Lu, Y., & Gao, S. (2021). Biochar effects on yield of cereal and legume crops using meta-analysis. Science of The Total Environment, 775, 145869. https://doi.org/10.1016/j.scitotenv.2021.145869
George, C., Wagner, M., Kücke, M., & Rillig, M. C. (2012). Divergent consequences of hydrochar in the plant–soil system: Arbuscular mycorrhiza, nodulation, plant growth and soil aggregation effects. Applied Soil Ecology, 59, 68–72. https://doi.org/10.1016/j.apsoil.2012.02.021
Hagemann, N., Joseph, S., Conte, P., Albu, M., Obst, M., Borch, T., Orsetti, S., Subdiaga, E., Behrens, S., & Kappler, A. (2017). Composting-derived organic coating on biochar enhances its affinity to nitrate. 10775. EGU General Assembly Conference Abstracts. https://ui.adsabs.harvard.edu/abs/2017EGUGA..1910775H
Jalal, F., Arif, M., Ahmad, I., & Murad, W. (2018). Increasing Farm Productivity and Soil Fertility on Sustainable Basis Through “Summer Gap” Utilization with Biochar and Legumes. Gesunde Pflanzen, 70(1), 45–53. https://doi.org/10.1007/s10343-017-0412-x
Janu, R., Mrlik, V., Ribitsch, D., Hofman, J., Sedláček, P., Bielská, L., & Soja, G. (2021). Biochar surface functional groups as affected by biomass feedstock, biochar composition and pyrolysis temperature. Carbon Resources Conversion, 4, 36–46. https://doi.org/10.1016/j.crcon.2021.01.003
Jindo, K., Mizumoto, H., Sawada, Y., Sanchez-Monedero, M. A., & Sonoki, T. (2014). Physical and chemical characterization of biochars derived from different agricultural residues. Biogeosciences, 11(23), 6613–6621. https://doi.org/10.5194/bg-11-6613-2014
Joseph, S., Kammann, C. I., Shepherd, J. G., Conte, P., Schmidt, H.-P., Hagemann, N., Rich, A. M., Marjo, C. E., Allen, J., Munroe, P., Mitchell, D. R. G., Donne, S., Spokas, K., & Graber, E. R. (2018). Microstructural and associated chemical changes during the composting of a high temperature biochar: Mechanisms for nitrate, phosphate and other nutrient retention and release. Science of The Total Environment, 618, 1210–1223. https://doi.org/10.1016/j.scitotenv.2017.09.200
Kammann, C. I., Schmidt, H.-P., Messerschmidt, N., Linsel, S., Steffens, D., Müller, C., Koyro, H.-W., Conte, P., & Joseph, S. (2015). Plant growth improvement mediated by nitrate capture in co-composted biochar. Scientific Reports, 5(1), 11080. https://doi.org/10.1038/srep11080
Kapoor, A., Sharma, R., Kumar, A., & Sepehya, S. (2022). Biochar as a means to improve soil fertility and crop productivity: A review. Journal of Plant Nutrition, 45(15), 2380–2388. https://doi.org/10.1080/01904167.2022.2027980
Küçükbayrak, S., & Kadioǧlu, E. (1989). Effect of pyrolysis on the proximate and ultimate analysis of lignite. Thermochimica Acta, 155, 1–6. https://doi.org/10.1016/0040-6031(89)87131-X
Kul, R., Arjumend, T., Ekinci, M., Yildirim, E., Turan, M., & Argin, S. (2021). Biochar as an organic soil conditioner for mitigating salinity stress in tomato. Soil Science and Plant Nutrition, 67(6), 693–706. https://doi.org/10.1080/00380768.2021.1998924
Kumari, S., Kumar, V., Kothari, R., & Kumar, P. (2022). Effect of supplementing biochar obtained from different wastes on biochemical and yield response of French bean (Phaseolus vulgaris L.): An experimental study. Biocatalysis and Agricultural Biotechnology, 43, 102432. https://doi.org/10.1016/j.bcab.2022.102432
Lehmann, J., Gaunt, J., & Rondon, M. (2006). Bio-char Sequestration in Terrestrial Ecosystems – A Review. Mitigation and Adaptation Strategies for Global Change, 11(2), 403–427. https://doi.org/10.1007/s11027-005-9006-5
Li, S., Harris, S., Anandhi, A., & Chen, G. (2019). Predicting biochar properties and functions based on feedstock and pyrolysis temperature: A review and data syntheses. Journal of Cleaner Production, 215, 890–902. https://doi.org/10.1016/j.jclepro.2019.01.106
Liu, Y., He, Z., & Uchimiya, M. (2015). Comparison of Biochar Formation from Various Agricultural By-Products Using FTIR Spectroscopy. Modern Applied Science, 9(4), p246. https://doi.org/10.5539/mas.v9n4p246
Manyà, J. J. (2012). Pyrolysis for Biochar Purposes: A Review to Establish Current Knowledge Gaps and Research Needs. Environmental Science & Technology, 46(15), 7939–7954. https://doi.org/10.1021/es301029g
Marahatta, Prof. Dr. A. B., Baral, G., & Dhital, K. (2024). FTIR Spectroscopic Studies on Hydration Propensities of the Preferentially Selected OPC Cements Manufactured in Nepal. International Journal of Progressive Sciences and Technologies (IJPSAT), 45(2 July,2024), 1–21.
Martinsen, V., Mulder, J., Shitumbanuma, V., Sparrevik, M., Børresen, T., & Cornelissen, G. (2014). Farmer-led maize biochar trials: Effect on crop yield and soil nutrients under conservation farming. Journal of Plant Nutrition and Soil Science, 177(5), 681–695. https://doi.org/10.1002/jpln.201300590
Nandiyanto, A. B. D., Oktiani, R., & Ragadhita, R. (2019). How to Read and Interpret FTIR Spectroscope of Organic Material. Indonesian Journal of Science and Technology, 4(1), 97. https://doi.org/10.17509/ijost.v4i1.15806
Nasiri, M., Andalibi, B., Khomari, S., Goli, E., Nasiri, S., El-Keblawy, A., & Mastinu, A. (2024). Enhancing salt stress tolerance in kidney beans: The synergistic effects of biochar and salicylic acid in arid and semi-arid regions. Plant Stress, 11, 100423. https://doi.org/10.1016/j.stress.2024.100423
Ndor, E., Jayeoba, J. O., Asadu, C. L. A., & Iheshiulo, E. M.-A. (2016). Growth, Nutrient Uptake and Dry Matter Yield of Maize (Zea Mays L) Grown in Soil Amended with Rice Husk and Sawdust Biochar. International Journal of Scientific Research in Agricultural Sciences, 3(3), 99–103. https://doi.org/10.12983/ijsras-2016-p0099-0103
Obia, A., Mulder, J., Martinsen, V., Cornelissen, G., & Børresen, T. (2016). In situ effects of biochar on aggregation, water retention and porosity in light-textured tropical soils. Soil and Tillage Research, 155, 35–44. https://doi.org/10.1016/j.still.2015.08.002
Pandit, N. R., Dahal, S., Shrestha, S., Vista, S. P., & Gautam, D. K. (2021). Biochar as an efficient soil enhancer to improve soil fertility and crop productivity in Nepal. Nepalese Journal of Agricultural Sciences, 2021, 21.
Pandit, N. R., Mulder, J., Hale, S. E., Schmidt, H. P., & Cornelissen, G. (2017). Biochar from “Kon Tiki” flame curtain and other kilns: Effects of nutrient enrichment and kiln type on crop yield and soil chemistry. PLOS ONE, 12(4), e0176378. https://doi.org/10.1371/journal.pone.0176378
Pandit, N. R., Sipkhan, P., Sharma, S. S., Dawadi, D., Vista, S. P., & Raut, P. (2024). Cattle-Urine-Enriched Biochar Enhances Soil Fertility, Nutrient Uptake, and Yield of Maize in a Low-Productive Soil. Nitrogen, 5(1), 16–27. https://doi.org/10.3390/nitrogen5010002
Pariyar, P., Kumari, K., Jain, M. K., & Jadhao, P. S. (2020). Evaluation of change in biochar properties derived from different feedstock and pyrolysis temperature for environmental and agricultural application. Science of The Total Environment, 713, 136433. https://doi.org/10.1016/j.scitotenv.2019.136433
Puga, A. P., Grutzmacher, P., Cerri, C. E. P., Ribeirinho, V. S., & Andrade, C. A. D. (2020). Biochar-based nitrogen fertilizers: Greenhouse gas emissions, use efficiency, and maize yield in tropical soils. Science of The Total Environment, 704, 135375. https://doi.org/10.1016/j.scitotenv.2019.135375
Rahayu, M., Nurmalasari, A. I., & Aini, N. N. (2022). Effect of various types and doses of biochar on hybrid maize growth. IOP Conference Series: Earth and Environmental Science, 1016(1), 012053. https://doi.org/10.1088/1755-1315/1016/1/012053
Rillig, M. C., Wagner, M., Salem, M., Antunes, P. M., George, C., Ramke, H.-G., Titirici, M.-M., & Antonietti, M. (2010). Material derived from hydrothermal carbonization: Effects on plant growth and arbuscular mycorrhiza. Applied Soil Ecology, 45(3), 238–242. https://doi.org/10.1016/j.apsoil.2010.04.011
Rondon, M. A., Lehmann, J., Ramírez, J., & Hurtado, M. (2007). Biological nitrogen fixation by common beans (Phaseolus vulgaris L.) increases with bio-char additions. Biology and Fertility of Soils, 43(6), 699–708. https://doi.org/10.1007/s00374-006-0152-z
Ronsse, F., van Hecke, S., Dickinson, D., & Prins, W. (2013). Production and characterization of slow pyrolysis biochar: Influence of feedstock type and pyrolysis conditions. GCB Bioenergy, 5(2), 104–115. https://doi.org/10.1111/gcbb.12018
Rutherford, D. W., Wershaw, R. L., Rostad, C. E., & Kelly, C. N. (2012). Effect of formation conditions on biochars: Compositional and structural properties of cellulose, lignin, and pine biochars. Biomass and Bioenergy, 46, 693–701. https://doi.org/10.1016/j.biombioe.2012.06.026
Schmidt, H., Pandit, B., Martinsen, V., Cornelissen, G., Conte, P., & Kammann, C. (2015). Fourfold Increase in Pumpkin Yield in Response to Low-Dosage Root Zone Application of Urine-Enhanced Biochar to a Fertile Tropical Soil. Agriculture, 5(3), 723–741. https://doi.org/10.3390/agriculture5030723
Song, W., & Guo, M. (2012). Quality variations of poultry litter biochar generated at different pyrolysis temperatures. Journal of Analytical and Applied Pyrolysis, 94, 138–145. https://doi.org/10.1016/j.jaap.2011.11.018
Steiner, C., Teixeira, W. G., Lehmann, J., Nehls, T., De Macêdo, J. L. V., Blum, W. E. H., & Zech, W. (2007). Long term effects of manure, charcoal and mineral fertilization on crop production and fertility on a highly weathered Central Amazonian upland soil. Plant and Soil, 291(1–2), 275–290. https://doi.org/10.1007/s11104-007-9193-9
Syahrinudin, S., Hartati, W., Sudarmadji, T., Krisdianto, N., & Ibrahim, I. (2019). Biochar enriched with organic fertilizer improves the survival and growth rate of Anthocepalus cadamba seedlings planted on degraded spodosols. Biodiversitas Journal of Biological Diversity, 20(12). https://doi.org/10.13057/biodiv/d201237
Tomczyk, A., Sokołowska, Z., & Boguta, P. (2020). Biochar physicochemical properties: Pyrolysis temperature and feedstock kind effects. Reviews in Environmental Science and Bio/Technology, 19(1), 191–215. https://doi.org/10.1007/s11157-020-09523-3
Van Zwieten, L., Kimber, S., Morris, S., Chan, K. Y., Downie, A., Rust, J., Joseph, S., & Cowie, A. (2010). Effects of biochar from slow pyrolysis of papermill waste on agronomic performance and soil fertility. Plant and Soil, 327(1–2), 235–246. https://doi.org/10.1007/s11104-009-0050-x
Wiedemeier, D. B., Abiven, S., Hockaday, W. C., Keiluweit, M., Kleber, M., Masiello, C. A., McBeath, A. V., Nico, P. S., Pyle, L. A., Schneider, M. P. W., Smernik, R. J., Wiesenberg, G. L. B., & Schmidt, M. W. I. (2015). Aromaticity and degree of aromatic condensation of char. Organic Geochemistry, 78, 135–143. https://doi.org/10.1016/j.orggeochem.2014.10.002
Zaitun, Z., Halim, A., Sa’dah, Y., & Cahyadi, R. (2022). Surface morphology properties of biochar feedstock for soil amendment. IOP Conference Series: Earth and Environmental Science, 951(1), 012034. https://doi.org/10.1088/1755-1315/951/1/012034
Zhang, X., Davidson, E. A., Mauzerall, D. L., Searchinger, T. D., Dumas, P., & Shen, Y. (2015). Managing nitrogen for sustainable development. Nature, 528(7580), 51–59. https://doi.org/10.1038/nature15743
Zhang, Y., Ma, Z., Zhang, Q., Wang, J., Ma, Q., Yang, Y., Luo, X., & Zhang, W. (2017). Comparison of the Physicochemical characteristics of bio-char pyrolyzed from moso bamboo and rice husk with different pyrolysis temperatures. BioResources, 12(3), 4652–4669. https://doi.org/10.15376/biores.12.3.4652-4669
Zhao, H., Xie, T., Xiao, H., & Gao, M. (2022). Biochar-Based fertilizer improved crop yields and N utilization efficiency in a maize–Chinese cabbage rotation system. Agriculture, 12(7), Article 7. https://doi.org/10.3390/agriculture12071030
Zhu, Z., Wei, G., Li, J., Qian, Q., & Yu, J. (2004). Silicon alleviates salt stress and increases antioxidant enzymes activity in leaves of salt-stressed cucumber (Cucumis sativus L.). Plant Science, 167(3), 527–533. https://doi.org/10.1016/j.plantsci.2004.04.020
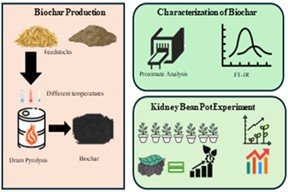
Published
How to Cite
Issue
Section
Copyright (c) 2024 Agriculture and Environmental Science Academy

This work is licensed under a Creative Commons Attribution-NonCommercial 4.0 International License.